![]() |
Sir J.J. Thomson
(18 Dec 1856 - 30 Aug 1940)
English physicist who investigated what were known as cathode rays, and discovered the electron (1897) and received the 1906 Nobel Prize for Physics.
|
Cathode Rays.
WEEKLY EVENING MEETING,
Friday, April 30, 1897
Sir Frederick Bramwell, Bart. D.C.L. .D. F.R.S. Honorary Secretary and Vice-President, in the Chair
Professor J. J. Thomson, M.A. LL.D. Sc.D. F.R.S.
[p.419] The first observer to leave any record of what are now known as the cathode rays seems to have been Plücker, who in 1859 observed the now well-known green phosphorescence on the glass in the neighborhood of the negative electrode. Plücker was the first physicist to make experiments on the discharge through a tube in a state anything approaching what we should now call a high vacuum. He owed the opportunity to do this to his fellow-townsman Geissler, who first made such vacua attainable. Plücker, who had made a very minute study of the effect of a magnetic field on the ordinary discharge which stretches from one terminal to the other, distinguished the discharge which produced the green phosphorescence from the ordinary discharge by the difference in its behavior when in a magnetic field. Plücker ascribed these phosphorescent patches to currents of electricity which went from the cathode to the walls of the tube and then for some reason or other retraced their steps.
The subject was next taken up by Plücker’s pupil, Hittorf, who greatly extended our knowledge of the subject, and to whom we owe the observation that a solid body placed between a pointed cathode and the walls of the tube cast a well defined shadow. This observation was extended by Goldstein, who found that a well marked, though not very sharply defined, shadow was cast by a small body placed near a cathode of considerable area. This was a very important observation, for it showed that the rays casting the shadow came in a definite direction from the cathode. If the cathode were replaced by a luminous disk of the same size, this disk would not cast a shadow of a small object placed near it, for though the object might intercept the rays which came out normally from the disk, yet enough light would be given out sideways from other parts of the disk to prevent the shadow being at all well marked. Goldstein seems to have been the first to advance the theory, which has attained a good deal of prevalence in Germany, that these cathode rays are transversal vibrations in the ether.
The physicist, however, who did more than any one else to direct attention to these rays was Mr. Crookes, whose experiments, by their beauty and importance, attracted the attention of all physicists to this [p.420] subject, and who not only greatly increased our knowledge of the properties of the rays, but by his application of them to radiant matter spectroscopy has rendered them most important agents in chemical research.
Recently a great renewal of interest in these rays has taken place, owing to the remarkable properties possessed by an offsprings of theirs, for the cathode rays are the parents of the Röntgen rays.
I shall confine myself this evening to endeavoring to give an account of some of the more recent investigations which have been made on the cathode rays. In the first place, when these rays fall on a substance they produce changes physical or chemical in the nature of the substance. In some cases this change is marked by a change in the color of the substance, as in the case of the chlorides of the alkaline metals. Goldstein found that these, when exposed to the cathode rays, changed color, the change, according to E. Wiedemann and Ebert, being due to the formation of a subchloride. Elster and Geitel have recently shown that these substances become photoelectric—i.e., acquire the power of discharging negative electricity under the action of light after exposure to the cathode rays. But though it is only in comparatively few cases that the change produced by the cathode rays shows itself in such a conspicuous way as by a change of color, there is a much more widely spread phenomenon, which shows the permanence of the effect produced by the impact of these rays. This is the phenomenon called by its discoverer, Prof. E. Wiedemann, thermoluminesceuce. Professor Wiedemann finds that if bodies are exposed to the cathode rays for some time, when the bombardment stops the substance resumes to all appearance its original condition. When, however, we heat the substance, we find that a change has taken place; for the substance now, when heated, becomes luminous at a comparatively low temperature, one far below that of incandescence. The substance retains this property for months after the exposure to the rays has ceased. The phenomenon of thermoluminescence is especially marked in bodies which are called by Van t’Hoff solid solutions. These are formed when two salts, one greatly in excess of the other, are simultaneously precipitated from a solution. Under these circumstances the connection between the salts seems of a more intimate character than that existing in a mechanical mixture. I have here a solid solution of CaSO4 with trace of MnSO4, and you will see that after exposure to the cathode rays it becomes luminous when heated. Another proof of the alteration produced by these rays is the fact, discovered by Crookes, that after glass has been exposed for a long time to the impact of these rays, the intensity of its phosphorescence is less than when the rays first began to fall upon it. This alteration lasts for a long time, certainly for months, and Mr. Crookes has shown that it is able to survive the heating up of the glass to allow of the remaking of the bulb. I will now leave the chemical effects produced by these rays and pass on to consider their behavior when in a magnetic field.
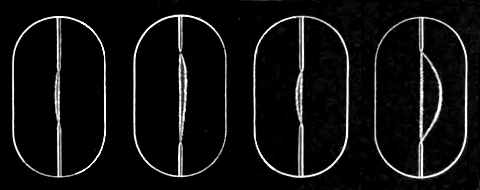
[p.421] First, let us consider for a moment the effect of magnetic force on the ordinary discharge between terminals at a pressure much higher than that at which the cathode rays begin to come off. I have here photographs (see Figs. 1 and 2) of the spark in a magnetic field. You see that when the discharge, which passes as a thin bright line between the terminals, is acted upon by the magnetic field it is pulled aside as a stretched string would be if acted upon by a force at right angles to its length. The curve is quite continuous, and though there may be gaps in the luminosity of the discharge, yet there are no breaks at such points in the curve, into which the discharge is bent by [p.422] a magnet. Again, if the discharge, instead of taking place between points passes between flat discs, the effect of the magnetic force is to move the sparks as a whole, the sparks keeping straight until their terminations reach the edges of the discs. The fine thread-like discharge is not much spread out by the action of the magnetic field. The appearance of the discharge indicates that when the discharge passes through the gas it manufactures out of the gas something stretching from terminal to terminal, which, unlike a gas, is capable of sustaining a tension. The amount of deflection produced, other circumstances being the same, depends on the nature of the gas. As the photographs (figs. 3 and 4) show, the deflection is very small in the case of hydrogen and very considerable in the case of carbonic acid; as a general rule it seems smaller in elementary than in compound gases.
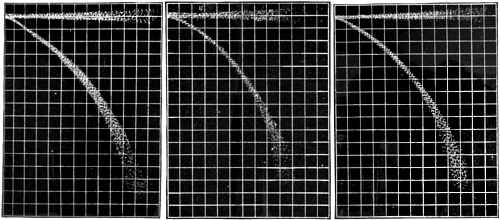
Fig. 6— Air.
Fig. 7— Carbonic Acid Gas (Ammeter, 12; Voltmeter, 1600).
Let us contrast the behavior of this kind of discharge under the action of a magnetic field with that of the cathode rays. I have here some photographs (Figs 5, 6 and 7) taken of a narrow beam formed by sending the cathode rays through a tube in which there was a plug with a slit in it, the plug being used as an anode and connected with the earth, these rays traversing a uniform magnetic field. The narrow beam spreads out under the action of the magnetic force into a broad fan-shaped luminosity in the gas. The luminosity in this fan is not uniformly distributed, but is condensed along certain lines. The phosphorescence produced when the rays reach the glass is also not uniformly distributed. It is much spread out, showing that the beam consists of rays which are not all deflected to the same extent [p.423] by the magnet. The luminous patch on the glass is crossed by bands along which the luminosity is very much greater than in the adjacent parts. These bright and dark bands are called by Birkeland, who first observed them, “the magnetic spectrum.” The brightest places [p.424] on the glass are by no means always the terminations of the brightest streaks of luminosity in the gas; in fact, in some cases a very bright spot on the glass is not connected with the cathode by any appreciable luminosity, though there is plenty of luminosity in other parts of the gas.
One very interesting point brought out by the photographs is that in a given magnetic field, with a given mean potential difference between the terminals, the path of the rays is independent of the nature of the gas. Photographs were taken of the discharge in hydrogen, air, carbonic acid, methyl iodide, i.e., in gases whose densities range from 1 to 70, and yet not only were the paths of the most deflected rays the same in all cases, but even the details, such as the distribution of the bright and dark spaces, were the same; in fact, the photographs could hardly be distinguished from one another. It is to be noted that the pressures were not the same; the pressures were adjusted until the menu potential difference was the same. When the pressure of the gas is lowered, the potential difference between the terminals increases, and the deflection of the rays produced by a magnet diminishes, or at any rate the deflection of the rays where the phosphorescence is a maximum diminishes. If an air break is inserted in the circuit an effect of the same kind is produced. In all the photographs of the cathode rays one sees indications of rays which stretch far into the bulb, but which are not deflected at all by a magnet. Though they stretch for some 2 or 3 inches, yet in none of these photographs do they actually reach the glass. In some experiments, however, I placed inside the tube a screen, near to the slit through which the cathode rays came, and found that no appreciable phosphorescence was produced when the non deflected rays struck the screen, while there was vivid phosphorescence at the places where the deflected rays struck the screen. These non deflected rays do not seem to exhibit any of the characteristics of cathode rays, and it seems possible that they are merely jets of uncharged luminous gas shot out through the slit from the neighborhood of the cathode by a kind of explosion when the discharge passes.
The curves described by the cathode rays in a uniform magnetic field are, very approximately at any rate, circular for a large part of their course. This is the path which would be described if the cathode rays marked the path of negatively electrified particles projected with great velocities from the neighborhood of the negative electrode. Indeed, all the effects produced by a magnet on these rays, and some of these are complicated, as, for example, when the rays are curled up into spirals under the action of a magnetic force, are in exact agreement with the consequences of this view.
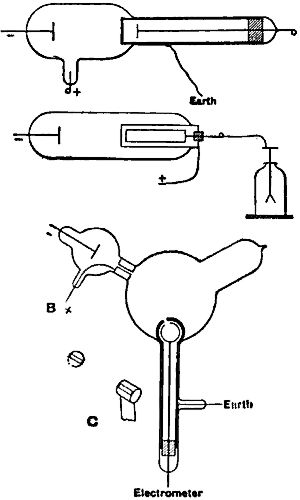
We can, moreover, show by direct experiment that a charge of negative electricity follows the course of the cathode rays. One way in which this has been done is by an experiment due to Perrin, the details of which are shown in the accompanying illustration (Fig. 8). In this experiment the rays are allowed to pass inside a metallic cylinder [p.425] through a small hole, and the cylinder, when these rays enter it, gets a negative charge, while if the rays are deflected by a magnet, so as to escape the hole, the cylinder remains without charge. It seems to me that to the experiment in this form it might be objected that, though the experiment shows that negatively electrified bodies are projected normally from the cathode and are deflected by a magnet, it does not show that when the cathode rays are deflected by a magnet the path of the electrified particles coincides with the path of the cathode rays. The supporters of the theory that these rays are waves in the ether might say, and indeed have said, that while they did not deny that electrified particles might be shot off from the cathode, these particles were, in their opinion, merely accidental accompaniments of the rays, and had no more to do with the rays than the bullet has with the flash of a rifle. The following modification of Perrin’s experiment is not, however, open to this objection: Two coaxial cylinders with slits cut in them, the outer cylinder being connected with earth, the inner with the electrometer, are placed in the discharge tube, but in such a position that the cathode rays do not fall upon them unless deflected by a magnet. By means of a magnet, however, we can deflect the cathode rays until they fall on the slit in the cylinder. If under these circumstances the cylinder gets a negative charge when the cathode rays fall on the slit, and remains uncharged unless they do so, we may conclude, I think, the stream of negatively electrified particles is an invariable accompaniment of the cathode rays. I will now try the experiment. You notice that when there is no magnetic force, though the rays do not fall on the cylinder, there is a slight deflection of the electrometer, [p.426] showing that it has acquired a small negative charge. This is, I think, due to the plug getting negatively charged under the torrent of negatively electrified particles from the cathode, and getting out cathode rays on its own account which have not come through the slit. I will now deflect the rays by a magnet, and you will see that at first there is little or no change in the deflection of the electrometer, but that when the rays reach the cylinder there is at once a great increase in the deflection, showing that the rays are pouring a charge of negative electricity into the cylinder. The deflection of the electrometer reaches a certain value and then stops and remains constant, though the rays continue to pour into the cylinder. This is due to the fact that the gas traversed by the cathode rays becomes a conductor of electricity, and thus, though the inner cylinder is perfectly insulated when the rays are not passing, yet as soon as the rays pass through the bulb the air between the inner cylinder and the outer one, which is connected with the earth, becomes a conductor, and the electricity escapes from the inner cylinder to the earth. For this reason the charge within the inner cylinder does not go on continually increasing. The cylinder settles into a state of equilibrium in which the rate at which it gains negative electricity from the rays is equal to the rate at which it loses it by conduction through the air. If we charge up the cylinder positively it rapidly loses its positive charge and acquires a negative one, while if we charge it up negatively it will leak if its initial negative potential is greater than its equilibrium value.
I have lately made some experiments which are interesting from the bearing they have on the charges carried by the cathode rays, as well as on the production of cathode rays outside the tube. The experiments are of the following kind: In the tube (Fig. 10) A and B are terminals. C is a long side tube into which a closed metallic cylinder fits lightly. This cylinder is made entirely of metal, except the end farthest from the terminals, which is stopped by an ebonite plug, perforated by a small hole so as to make the pressure inside the cylinder equal to that in the discharge tube. Inside the cylinder there is a metal disk supported by a metal rod which passes through the ebonite plug and is connected with an electrometer, the wires making this connection being surrounded by tubes connected with the earth so as to screen off electrostatic induction. If the end of the cylinder is made of thin aluminum about 1/20th of a millimetre thick, and a discharge sent between the terminals, A being the cathode, then at pressures far higher than those at which the cathode rays come off, the disk inside the cylinder acquires a positive charge; and if it is charged up independently the charge leaks away, and it leaks more rapidly when the disk is charged negatively than when it is charged positively. There is, however, a leak in both cases, showing that conduction has taken place through the gas between the cylinder and the disk. As the pressure in the tube is diminished the positive charge on the disk diminishes until it becomes unappreciable. The [p.427] leak from the disk, when it is charged still continues, and is now equally rapid, whether the original charge on the disk is positive or negative. When the pressure falls so low that cathode rays begin to fall on the end of the cylinder, then the disk acquires a negative charge, and the leak from the disk is more rapid when it is charged positively than when it is charged negatively. If the cathode rays are pulled off the end of the cylinder by a magnet, then the negative charge on the disk and the rate of leak from the disk when it is positively charged is very much diminished. A very interesting point is that these effects, due to the cathode rays, are observed behind comparatively thick walls. I have here a cylinder whose base is brass about 1 mm. thick, and yet when this is exposed to the cathode rays the disk behind it gets a negative charge, and leaks if charged positively. The effect is small, compared with that in the cylinder with the thin aluminum base, but is quite appreciable. With the cylinder with the thick end I have never been able to observe any effect at the higher pressure when no cathode rays were coming off. The effect with the cylinder with the thin end was observed when the discharge was produced by a large number of small storage cells, as well as when it was produced by an induction coil.
It would seem from this experiment that the incidence of the cathode rays on a brass plate as much as 1 mm. thick and connected with the earth can put a rarefied gas shielded by the plate into a condition in which it can conduct electricity, and that a body placed behind this screen gets a negative charge, so that the side of the brass away from the cathode rays acts itself like a cathode, though kept permanently to earth. In the case of the thick brass the effect seems much more likely to be due to a sudden change in the potential of the outer cylinder at the places where the rays strike rather than to the penetration of any kinds of waves or rays. If the discharge in the tube was perfectly continuous the potential of the outer cylinder would be constant, and since it is connected to earth by a wire through which no considerable current flows, the potential must be approximately that of the earth. The discharge there can not be continuous; the negative charge must come in gusts against the ends of the cylinder, coming so suddenly that the electricity has no time to distribute itself over the cylinder so as to shield off the inside from [p.428] the electrostatic action of the cathode rays; this force penetrates the cylinder and produces a discharge of electricity from the far side of the brass.
Another effect which I believe is due to the negative electrification carried by the rays is the following: In a very highly exhausted tube provided with a metal plug I have sometimes observed, after the coil has been turned off, bright patches on the glass. These are deflected by a magnet and seem to be caused by the plug getting such a large negative charge that the negative electricity continues to stream from it after the coil is stopped.
An objection sometimes urged against the view that these cathode rays consist of charged particles is that they are not deflected by an electrostatic force. If, for example, we make, as Hertz did, the rays pass between plates connected with a battery, so that au electrostatic force acts between these plates, the cathode ray is able to traverse this space without being deflected one way or the other. We must remember, however, that the cathode rays when they pass through a gas make it a conductor, so that the gas, acting like a conductor, screens off the electric force from the charged particle, and when the plates are immersed in the gas, and a definite potential difference established between the plates, the conductivity of the gas close to the cathode rays is probably enormously greater than the average conductivity of the gas between the plates, and the potential gradient on the cathode rays is therefore very small compared with the average potential gradient. We can, however, produce electrostatic results if we put the conductors which are to deflect the rays in the dark space next the cathode. I have here a tube in which, inside the dark space next the cathode, two conductors are inserted; the cathode rays start from the cathode, and have to pass between these conductors; if, now, I connect one of these conductors to earth there is a decided deflection of the cathode rays, while if I connect the other electrode to earth there is a deflection in the opposite direction. I ascribe this deflection to the gas in the dark space either not being a conductor at all, or if a conductor, a poor one compared to the gas in the main body of the tube.
Goldstein has shown that if a tube is furnished with two cathodes, when the rays from one cathode pass near the other they are repelled from it. This is just what would happen if the dark space round the electrode were an insulator, and so able to transmit electrostatic attractions or repulsions. To show that the gas in the dark space differs in its properties from the rest of the gas, I will try the following experiment. I have here two spherical bulbs connected together by a glass tube; one of these bulbs is small, the other large; they each contain a cathode, and the pressure of the gas is such that the dark space round the cathode in the small bulb completely fills the bulb, while that round the one in the larger bulb does not extend to the walls of the bulb. The two bulbs are wound with wire, which connects the outsides of two Leyden jars; the insides of these jars [p.429] are connected with the terminals of a Wimshurst machine. When sparks pass between these terminals currents pass through the wire which induce currents in the bulbs, and cause a ring discharge to pass through them. Things are so arranged that the ring is faint in the larger bulb, bright in the smaller one. On making the wires in these bulbs cathodes, however, the discharge in the small bulb, which is filled by the dark space, is completely stopped, while that in the larger one becomes brighter. Thus the gas in the dark space is changed, and in the opposite way from that in the rest of the tube. It is remarkable that when the coil is stopped the ring discharge on both bulbs stops, and it is some time before it starts again.
The deflection excited on each other by two cathodic streams would seem to have a great deal to do with the beautiful phosphorescent figures which Goldstein obtained by using cathodes of different shapes. I have here two bulbs containing cathodes shaped like a cross. They are curved and of the same radius as the bulb, so that if the rays came off these cathodes normally the phosphorescent picture ought to be a cross of the same size as the cathode. You see that in one of the bulbs the image of the cross consists of two large sectors at right angles to each other, bounded by bright lines, and in the other, which is at a lower pressure, the geometrical image of the cross instead of being bright is dark, while the luminosity occupies the space between the arms of the cross.
So far I have only considered the behavior of the cathode rays inside the bulb, but Lenard has been able to get these rays outside the tube. To do this he let the rays fall on a window in the tube, made of thin aluminum about one 1/100th of a millimetre thick, and he found that from this window there proceeded in all directions rays which were deflected by a magnet, and which produced phosphorescence when they fell upon certain substances, notably upon tissue paper soaked in a solution of pentadekaparalolylketon. The very thin aluminum is difficult to get, and Mr. McClelland has found that if it is not necessary to maintain the vacuum for a long time oiled silk answers admirably for a window. As the window is small, the phosphorescent patch produced by it is not bright, so that I will show instead the other property of the cathode rays—that of carrying with them a negative charge. I will place this cylinder in front of the hole, connect it with the electrometer, turn on the rays, and you will see the cylinder gets a negative charge. Indeed, this charge is large enough to produce the well-known negative figures when the rays fall on a piece of ebonite which is afterwards dusted with a mixture of red lead and sulphur.
From the experiments with the closed cylinder we have seen that when the negative rays come up to a surface even as thick as a millimetre the opposite side of that surface acts like a cathode and gives off the cathodic rays, and from this point of view we can understand the very interesting result of Lenard that the magnetic deflection of [p.430] the rays outside the tube is independent of the density and chemical composition of the gas outside the tube, though it varies very much with the pressure of the gas inside the tube. The cathode rays could be started by an electric impulse, which would depend entirely on what was going on inside the tube. Since the impulse is the same, the momentum acquired by the particles outside would be the same, and, as the curvature of the path only depends on the momentum, the path of these particles outside the tube would only depend on the state of affairs inside the tube.
The investigation by Lenard on the absorption of these rays shows that there is more in his experiment than is covered by this consideration. Lenard measured the distance these rays would have to travel before the intensity of the rays fell to one-half their original value. The results are given in the following table:—
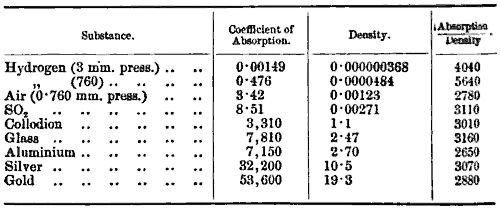
We see that though the densities and the coefficient of absorption vary enormously, yet the ratio of the two varies very little, and the results justify, I think, Lenard’s conclusion that the distance through which these rays travel only depends on the density of the substance— that is, the mass of matter per unit volume—and not upon the nature of the matter.
These numbers raise a question which I have not yet touched upon, and that is the size of the carriers of the electric charge. Are they or are they not the dimensions of ordinary matter?
We see from Lenard’s table that a cathode ray can travel through air at atmospheric pressure a distance of about half a centimetre before the brightness of the phosphorescence falls to about one-half of its original value. Now the mean free path of the molecule of air at this pressure is about 10–5 cm., and if a molecule of air were projected it would lose half its momentum in a space comparable with the mean free path. Even if we suppose that it is not the same molecule that is carried, the effect of the obliquity of the collisions would reduce the momentum to one half in a short multiple of that path.
Thus, from Lenard’s experiments on the absorption of the rays outside the tube, it follows, on the hypothesis that the cathode rays [p.431] are charged particles, moving with high velocities, that the size of the carriers must be small compared with the dimensions of ordinary atoms or molecules. The assumption of a state of matter more finely subdivided than the atom of an element is a somewhat startling one; but an hypothesis that would involve somewhat similar consequences, viz, that the so-called elements are compounds of some primordial element, has been put forward from time to time by various chemists. Thus, Prout believed that the atoms of all the elements were built up of atoms of hydrogen, and Mr. Norman Lockyer has advanced weighty arguments, founded on spectroscopic consideration, in favor of the composite nature of the elements.
Let us trace the consequence of supposing that the atoms of the elements are aggregations of very small particles, all similar to each other. We shall call such particles corpuscles, so that the atoms of the ordinary elements are made up of corpuscles and holes, the holes being predominant. Let us suppose that at the cathode some of the molecules of the gas get split up into these corpuscles, and that these, charged with negative electricity and moving at a high velocity, form the cathode rays. The distance these rays would travel before losing a given fraction of their momentum would be proportional to the mean free path of the corpuscles. Now, the things these corpuscles strike against are other corpuscles, and not against the molecules as a whole; they are supposed to be able to thread their way between the interstices in the molecule. Thus the mean free path would be proportional to the number of these corpuscles; and, therefore, since each corpuscle has the same mass to the mass of unit volume—that is, to the density of the substance, whatever be its chemical nature or physical state, the mean free path, and therefore the coefficient of absorption, would depend only on the density. This is precisely Lenard’s result.
We see, too, on this hypothesis, why the magnetic deflection is the same inside the tube, whatever be the nature of the gas, for the carriers of the charge are the corpuscles, and these are the same whatever gas be used. All the carriers may not be reduced to their lowest dimensions; some may be aggregates of two or more corpuscles; these would be differently deflected from the single corpuscle; thus we should get the magnetic spectrum.
I have endeavored by the following method to get a measurement of the ratio of the mass of these corpuscles to the charge carried by them. A double cylinder with slits in it, such as that used in a former experiment, was placed in front of a cathode which was curved so as to focus to some extent the cathode rays on the slit; behind the slit, in the inner cylinder, a thermal junction was placed which covered the opening so that all the rays which entered the slit struck against the junction, the junction got heated, and knowing the thermal capacity of the junction, we could get the mechanical equivalent of the heat communicated to it. The deflection of the electrometer gave the charge which entered the cylinder. [p.432] Thus, if there are N particles entering the cylinder each with a charge e, and Q is the charge inside the cylinder,
N e = Q.
The kinetic energy of these
½ N m v2
where W is the mechanical equivalent of the heat given to the thermal junction. By measuring the curvature of the rays for a magnetic field, we get
mv/e = I.
Thus
m/e = ½ Q I2 / W.
In an experiment made at a very low pressure, when the rays were kept on for about one second, the charge was sufficient to raise a capacity of 1·5 microfarads to a potential of 16 volts. Thus
Q = 2·4 x l0–6.
The temperature of the thermo junction, whose thermal capacity was 0·005 was raised 3·3° C. by the impact of the rays, thus
W = 3·3 x 0·005 x 4·2 x 107 = 6·3 x 105.
The value of I was 280, thus
m / e = 1·6 x 10–7.
This is very small compared with the value 10–4 for the ratio of the mass of an atom of hydrogen to the charge carried by it. If the result stood by itself we might think that it was probable that e was greater than the atomic charge of atom rather than that m was less than the mass of a hydrogen atom. Taken, however, in conjunction with Lenard’s results for the absorption of the cathode rays, these numbers seem to favor the hypothesis that the carriers of the charges are smaller than the atoms of hydrogen.
It is interesting to notice the value of e/m, which we have found, from the cathode rays, is of the same order as the value of 10–7 deduced by Zeeman from his experiments on the effect of a magnetic field on the period of the sodium light.
[J. J. T.]
- Science Quotes by Sir J.J. Thomson.
- 18 Dec - short biography, births, deaths and events on date of Thomson's birth.
- Becquerel Rays - by J.J. Thomson in Harper’s Magazine (Jan 1903).
- J.J. Thompson And The Discovery Of The Electron, by E. A. Davis and Isabel Falconer. - book suggestion.
- Booklist for John Joseph Thomson.