X-ray Quotes (43 quotes)
XRay Quotes, X Ray Quotes
XRay Quotes, X Ray Quotes
[Our work on the structure of DNA] was fairly fast, but you know, we were lucky. One must remember, it was based on the X-ray work done here in London started off by Morris Wilkins and carried on by Rosalind Franklin, and we wouldn’t have got to the stage of at least having a molecular model, if it hadn't been for their work.
Quoted and cited from BBC radio (1999) in transcript of Australian ABC radio program PM (30 Jul 2004) on the ABC website.
Every teacher certainly should know something of non-euclidean geometry. Thus, it forms one of the few parts of mathematics which, at least in scattered catch-words, is talked about in wide circles, so that any teacher may be asked about it at any moment. … Imagine a teacher of physics who is unable to say anything about Röntgen rays, or about radium. A teacher of mathematics who could give no answer to questions about non-euclidean geometry would not make a better impression.
On the other hand, I should like to advise emphatically against bringing non-euclidean into regular school instruction (i.e., beyond occasional suggestions, upon inquiry by interested pupils), as enthusiasts are always recommending. Let us be satisfied if the preceding advice is followed and if the pupils learn to really understand euclidean geometry. After all, it is in order for the teacher to know a little more than the average pupil.
On the other hand, I should like to advise emphatically against bringing non-euclidean into regular school instruction (i.e., beyond occasional suggestions, upon inquiry by interested pupils), as enthusiasts are always recommending. Let us be satisfied if the preceding advice is followed and if the pupils learn to really understand euclidean geometry. After all, it is in order for the teacher to know a little more than the average pupil.
In George Edward Martin, The Foundations of Geometry and the Non-Euclidean Plane (1982), 72.
A great advantage of X-ray analysis as a method of chemical structure analysis is its power to show some totally unexpected and surprising structure with, at the same time, complete certainty.
In 'X-ray Analysis of Complicated Molecules', Nobel Lecture (11 Dec 1964). In Nobel Lectures: Chemistry 1942-1962 (1964), 83.
A plain, reasonable working man supposes, in the old way which is also the common-sense way, that if there are people who spend their lives in study, whom he feeds and keeps while they think for him—then no doubt these men are engaged in studying things men need to know; and he expects of science that it will solve for him the questions on which his welfare, and that of all men, depends. He expects science to tell him how he ought to live: how to treat his family, his neighbours and the men of other tribes, how to restrain his passions, what to believe in and what not to believe in, and much else. And what does our science say to him on these matters?
It triumphantly tells him: how many million miles it is from the earth to the sun; at what rate light travels through space; how many million vibrations of ether per second are caused by light, and how many vibrations of air by sound; it tells of the chemical components of the Milky Way, of a new element—helium—of micro-organisms and their excrements, of the points on the hand at which electricity collects, of X rays, and similar things.
“But I don't want any of those things,” says a plain and reasonable man—“I want to know how to live.”
It triumphantly tells him: how many million miles it is from the earth to the sun; at what rate light travels through space; how many million vibrations of ether per second are caused by light, and how many vibrations of air by sound; it tells of the chemical components of the Milky Way, of a new element—helium—of micro-organisms and their excrements, of the points on the hand at which electricity collects, of X rays, and similar things.
“But I don't want any of those things,” says a plain and reasonable man—“I want to know how to live.”
In 'Modern Science', Essays and Letters (1903), 221-222.
As a scientist Miss [Rosalind] Franklin was distinguished by extreme clarity and perfection in everything she undertook. Her photographs are among the most beautiful X-ray photographs of any substance ever taken.
In his Obituary for Rosalind Franklin, Nature, 1958, 182, 154. As given in Andrew Brown, J.D. Bernal: The Sage of Science (2005), 359.
As the nineteenth century drew to a close, scientists could reflect with satisfaction that they had pinned down most of the mysteries of the physical world: electricity, magnetism, gases, optics, acoustics, kinetics and statistical mechanics … all had fallen into order before them. They had discovered the X ray, the cathode ray, the electron, and radioactivity, invented the ohm, the watt, the Kelvin, the joule, the amp, and the little erg.
A Short History of Nearly Everything. In Clifford A. Pickover, Archimedes to Hawking: Laws of Science and the Great Minds Behind Them (2008), 172.
But in its [the corpuscular theory of radiation] relation to the wave theory there is one extraordinary and, at present, insoluble problem. It is not known how the energy of the electron in the X-ray bulb is transferred by a wave motion to an electron in the photographic plate or in any other substance on which the X-rays fall. It is as if one dropped a plank into the sea from the height of 100 ft. and found that the spreading ripple was able, after travelling 1000 miles and becoming infinitesimal in comparison with its original amount, to act upon a wooden ship in such a way that a plank of that ship flew out of its place to a height of 100 ft. How does the energy get from one place to the other?
'Aether Waves and Electrons' (Summary of the Robert Boyle Lecture), Nature, 1921, 107, 374.
By research in pure science I mean research made without any idea of application to industrial matters but solely with the view of extending our knowledge of the Laws of Nature. I will give just one example of the ‘utility’ of this kind of research, one that has been brought into great prominence by the War—I mean the use of X-rays in surgery. Now, not to speak of what is beyond money value, the saving of pain, or, it may be, the life of the wounded, and of bitter grief to those who loved them, the benefit which the state has derived from the restoration of so many to life and limb, able to render services which would otherwise have been lost, is almost incalculable. Now, how was this method discovered? It was not the result of a research in applied science starting to find an improved method of locating bullet wounds. This might have led to improved probes, but we cannot imagine it leading to the discovery of X-rays. No, this method is due to an investigation in pure science, made with the object of discovering what is the nature of Electricity. The experiments which led to this discovery seemed to be as remote from ‘humanistic interest’ —to use a much misappropriated word—as anything that could well be imagined. The apparatus consisted of glass vessels from which the last drops of air had been sucked, and which emitted a weird greenish light when stimulated by formidable looking instruments called induction coils. Near by, perhaps, were great coils of wire and iron built up into electro-magnets. I know well the impression it made on the average spectator, for I have been occupied in experiments of this kind nearly all my life, notwithstanding the advice, given in perfect good faith, by non-scientific visitors to the laboratory, to put that aside and spend my time on something useful.
In Speech made on behalf of a delegation from the Conjoint Board of Scientific Studies in 1916 to Lord Crewe, then Lord President of the Council. In George Paget Thomson, J. J. Thomson and the Cavendish Laboratory in His Day (1965), 167-8.
From the intensity of the spots near the centre, we can infer that the protein molecules are relatively dense globular bodies, perhaps joined together by valency bridges, but in any event separated by relatively large spaces which contain water. From the intensity of the more distant spots, it can be inferred that the arrangement of atoms inside the protein molecule is also of a perfectly definite kind, although without the periodicities characterising the fibrous proteins. The observations are compatible with oblate spheroidal molecules of diameters about 25 A. and 35 A., arranged in hexagonal screw-axis. ... At this stage, such ideas are merely speculative, but now that a crystalline protein has been made to give X-ray photographs, it is clear that we have the means of checking them and, by examining the structure of all crystalline proteins, arriving at a far more detailed conclusion about protein structure than previous physical or chemical methods have been able to give.
'X-Ray Photographs of Crystalline Pepsin', Nature (1934), 133, 795.
Great discoveries are made accidentally less often than the populace likes to think.
Referring to the accidental discovery of X-rays, in A History of Science and Its Relations with Philosophy and Religion (1931), 382.
I heard … xenon was a good anesthesia. … I thought, “How can xenon, which doesn’t form any chemical compounds, serve as a general anesthetic? … I lay awake at night for a few minutes before going to sleep, and during the next couple of weeks each night I would think, “…how do anesthetic agents work?" Then I forgot to do it after a while, but I’d trained my unconscious mind to keep this question alive and to call [it] to my consciousness whenever a new idea turned up…. So seven years went by. [One day I] put my feet up on the desk and started reading my mail, and here was a letter from George Jeffrey … an x-ray crystallographer, on his determination of the structure of a hydrate crystal. Immediately I sat up, took my feet off the desk, and said, “I understand anesthesia!” … I spent a year [and] determined the structure of chloroform hydrate, and then I wrote my paper published in June of 1961.
Interview with George B. Kauffman and Laurie M. Kauffman, in 'Linus Pauling: Reflections', American Scientist (Nov-Dec 1994), 82, No. 6, 522-523.
I should not like to leave an impression that all structural problems can be settled by X-ray analysis or that all crystal structures are easy to solve. I seem to have spent much more of my life not solving structures than solving them.
In 'X-ray Analysis of Complicated Molecules', Nobel Lecture (11 Dec 1964). In Nobel Lectures: Chemistry 1942-1962 (1964), 88.
I used to say the evening that I developed the first x-ray photograph I took of insulin in 1935 was the most exciting moment of my life. But the Saturday afternoon in late July 1969, when we realized that the insulin electron density map was interpretable, runs that moment very close.
'X-rays and the Structure of Insulin', British Medical Journal (1971), 4, 449.
I was working with a Crookes tube covered by a shield of black cardboard. A piece of barium platino-cyanide paper lay on the bench there. I had been passing a current through the tube, and I noticed a peculiar black line across the paper. …
The effect was one which could only be produced in ordinary parlance by the passage of light. No light could come from the tube because the shield which covered it was impervious to any light known even that of the electric arc. …
I did not think; I investigated. …
I assumed that the effect must have come from the tube since its character indicated that it could come from nowhere else. … It seemed at first a new kind of invisible light. It was clearly something new something unrecorded. …
There is much to do, and I am busy, very busy. [Describing to a journalist the discovery of X-rays that he had made on 8 Nov 1895.]
The effect was one which could only be produced in ordinary parlance by the passage of light. No light could come from the tube because the shield which covered it was impervious to any light known even that of the electric arc. …
I did not think; I investigated. …
I assumed that the effect must have come from the tube since its character indicated that it could come from nowhere else. … It seemed at first a new kind of invisible light. It was clearly something new something unrecorded. …
There is much to do, and I am busy, very busy. [Describing to a journalist the discovery of X-rays that he had made on 8 Nov 1895.]
In H.J.W. Dam in 'The New Marvel in Photography", McClure's Magazine (Apr 1896), 4:5, 413.
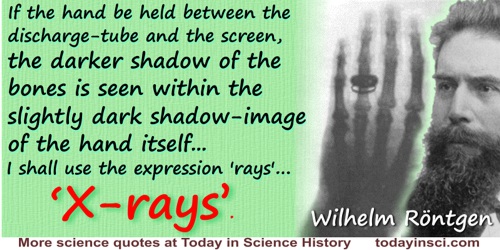
If the hand be held between the discharge-tube and the screen, the darker shadow of the bones is seen within the slightly dark shadow-image of the hand itself… For brevity’s sake I shall use the expression “rays”; and to distinguish them from others of this name I shall call them “X-rays”.
From 'On a New Kind of Rays' (1895). In Herbert S. Klickstein, Wilhelm Conrad Rontgen: On a New Kind of Rays, A Bibliographic Study (1966), 4.
In 1945 J.A. Ratcliffe … suggested that I [join his group at Cavendish Laboratory, Cambridge] to start an investigation of the radio emission from the Sun, which had recently been discovered accidentally with radar equipment. … [B]oth Ratcliffe and Sir Lawrence Bragg, then Cavendish Professor, gave enormous support and encouragement to me. Bragg’s own work on X-ray crystallography involved techniques very similar to those we were developing for “aperture synthesis,” and he always showed a delighted interest in the way our work progressed.
From Autobiography in Wilhelm Odelberg (ed.), Les Prix Nobel en 1974/Nobel Lectures (1975)
In describing a protein it is now common to distinguish the primary, secondary and tertiary structures. The primary structure is simply the order, or sequence, of the amino-acid residues along the polypeptide chains. This was first determined by Sanger using chemical techniques for the protein insulin, and has since been elucidated for a number of peptides and, in part, for one or two other small proteins. The secondary structure is the type of folding, coiling or puckering adopted by the polypeptide chain: the a-helix structure and the pleated sheet are examples. Secondary structure has been assigned in broad outline to a number of librous proteins such as silk, keratin and collagen; but we are ignorant of the nature of the secondary structure of any globular protein. True, there is suggestive evidence, though as yet no proof, that a-helices occur in globular proteins, to an extent which is difficult to gauge quantitatively in any particular case. The tertiary structure is the way in which the folded or coiled polypeptide chains are disposed to form the protein molecule as a three-dimensional object, in space. The chemical and physical properties of a protein cannot be fully interpreted until all three levels of structure are understood, for these properties depend on the spatial relationships between the amino-acids, and these in turn depend on the tertiary and secondary structures as much as on the primary. Only X-ray diffraction methods seem capable, even in principle, of unravelling the tertiary and secondary structures.
Co-author with G. Bodo, H. M. Dintzis, R. G. Parrish, H. Wyckoff, and D. C. Phillips
Co-author with G. Bodo, H. M. Dintzis, R. G. Parrish, H. Wyckoff, and D. C. Phillips
'A Three-Dimensional Model of the Myoglobin Molecule Obtained by X-ray Analysis', Nature (1958) 181, 662.
In my own field, x-ray crystallography, we used to work out the structure of minerals by various dodges which we never bothered to write down, we just used them. Then Linus Pauling came along to the laboratory, saw what we were doing and wrote out what we now call Pauling's Rules. We had all been using Pauling's Rules for about three or four years before Pauling told us what the rules were.
In The Extension of Man (1972), 116.
In the X-ray laboratory we are exposed, not only to the direct action of the rays, but to the effects of ionized air. This may be proved by hanging a charged silk tassel anywhere in the room. It will suddenly collapse when the current is turned on through the focus tube.
In 'Protection in X-Ray Work', Archives of the Roentgen Ray (July 1905), 10, No. 2, 38. [Note that this concern for protection, written in 1905, comes within 10 years of the discovery of X-Rays in 1895. —Webmaster]
Knowing what we know from X-ray and related studies of the fibrous proteins, how they are built from long polypeptide chains with linear patterns drawn to a grand scale, how these chains can contract and take up different configurations by intramolecular folding, how the chain- groups are penetrated by, and their sidechains react with, smaller co-operating molecules, and finally how they can combine so readily with nucleic acid molecules and still maintain the fibrous configuration, it is but natural to assume, as a first working hypothesis at least, that they form the long scroll on which is written the pattern of life. No other molecules satisfy so many requirements.
William Thomas Astbury and Florence O. Bell. 'Some Recent Developments in the X-Ray Study of Proteins and Related Structures', Cold Spring Harbor Symposia on Quantitative Biology, 1938, 6, 1144.
Over the last century, physicists have used light quanta, electrons, alpha particles, X-rays, gamma-rays, protons, neutrons and exotic sub-nuclear particles for this purpose [scattering experiments]. Much important information about the target atoms or nuclei or their assemblage has been obtained in this way. In witness of this importance one can point to the unusual concentration of scattering enthusiasts among earlier Nobel Laureate physicists. One could say that physicists just love to perform or interpret scattering experiments.
Nobel Banquet Speech (10 Dec 1994), in Tore Frängsmyr (ed.), Les Prix Nobel. The Nobel Prizes 1994 (1995).
Pauling was shocked by the freedom with which the X-ray crystallographers of the time, including particularly Astbury, played with the intimate chemical structure of their models. They seemed to think that if the atoms were arranged in the right order and about the right distance apart, that was all that mattered, that no further restrictions need to be put on them.
Quoted by John Law in 'The Case of X-ray Protein Crystallography', collected in Gerard Lemaine (ed.), Perspectives on the Emergence of Scientific Disciplines, 1976, 140.
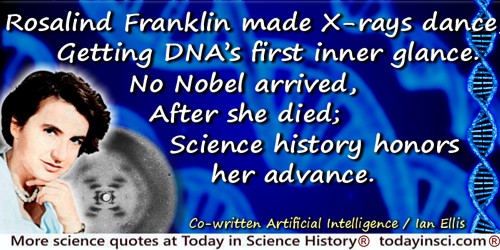
Rosalind Franklin made X-rays dance,
Getting DNA’s first inner glance.
No Nobel arrived,
After she died;
Science history honors her advance.
Getting DNA’s first inner glance.
No Nobel arrived,
After she died;
Science history honors her advance.
Limerick co-written by Artificial Intelligence and Ian Ellis. The ChatGTP limerick before edits by Webmaster was: “In labs with X-rays she would dance, / Gave DNA its first real glance, / No Nobel did arrive, / But her science thrives, / In history, she took a bold stance.
Science is something we depend on all the time. If I develop a pain in the chest I must take an X-ray. But what if the radiation from the X-ray causes me deeper problems? Before I know it. I’m going in for surgery. Naturally, while they’re giving me oxygen an intern decides to light up a cigarette. The next thing you know I’m rocketing over the World Trade Center in bed clothes. Is this science?
In 'My Speech to the Graduates', Side Effects (1986), 82.
Since the stomach gives no obvious external sign of its workings, investigators of gastric movements have hitherto been obliged to confine their studies to pathological subjects or to animals subjected to serious operative interference. Observations made under these necessarily abnormal conditions have yielded a literature which is full of conflicting statements and uncertain results. The only sure conclusion to be drawn from this material is that when the stomach receives food, obscure peristaltic contractions are set going, which in some way churn the food to a liquid chyme and force it into the intestines. How imperfectly this describes the real workings of the stomach will appear from the following account of the actions of the organ studied by a new method. The mixing of a small quantity of subnitrate of bismuth with the food allows not only the contractions of the gastric wall, but also the movements of the gastric contents to be seen with the Röntgen rays in the uninjured animal during normal digestion.
In 'The Movements of the Stomach Studied by Means of the Röntgen Rays,' American Journal of Physiology (1898), 1, 359-360.
Sodium thymonucleate fibres give two distinct types of X-ray diagram … [structures A and B]. The X-ray diagram of structure B (see photograph) shows in striking manner the features characteristic of helical structures, first worked out in this laboratory by Stokes (unpublished) and by Crick, Cochran and Vand2. Stokes and Wilkins were the first to propose such structures for nucleic acid as a result of direct studies of nucleic acid fibres, although a helical structure had been previously suggested by Furberg (thesis, London, 1949) on the basis of X-ray studies of nucleosides and nucleotides.
While the X-ray evidence cannot, at present, be taken as direct proof that the structure is helical, other considerations discussed below make the existence of a helical structure highly probable.
While the X-ray evidence cannot, at present, be taken as direct proof that the structure is helical, other considerations discussed below make the existence of a helical structure highly probable.
From Rosalind Franklin and R. G. Gosling,'Molecular Configuration in Sodium Thymonucleate', Nature (25 Apr 1953), 171, No. 4356, 740.
The fact that the general incidence of leukemia has doubled in the last two decades may be due, partly, to the increasing use of x-rays for numerous purposes. The incidence of leukemia in doctors, who are likely to be so exposed, is twice that of the general public. In radiologists … the incidence is ten times greater.
(1965). In Isaac Asimov’s Book of Science and Nature Quotations (1988), 233.
The great object is to find the theory of the matter [of X-rays] before anyone else, for nearly every professor in Europe is now on the warpath.
In Letter to Mary Newton (28 Jan 1896), collected in Arthur Stewart Eve, Rutherford: Being the Life and Letters of the Rt. Hon. Lord Rutherford, O.M. (1939), 25.
The present rate of progress [in X-ray crystallography] is determined, not so much by the lack of problems to investigate or the limited power of X-ray analysis, as by the restricted number of investigators who have had a training in the technique of the new science, and by the time it naturally takes for its scientific and technical importance to become widely appreciated.
Concluding remark in Lecture (1936) on 'Forty Years of Crystal Physics', collected in Needham and Pagel (eds.) in Background to Modern Science: Ten Lectures at Cambridge Arranged by the History of Science Committee, (1938), 89.
The question of protection against the noxious action of the X rays appears to be assuming more and more importance, as we begin to recognise the searching nature of their action on the deep-seated tissues and their far-reaching therapeutic effect on the internal organs.
In 'Protection in X-Ray Work', Archives of the Roentgen Ray (July 1905), 10, No. 2, 38. [Note that this concern for protection, written in 1905, comes within 10 years of the discovery of X-Rays in 1895. —Webmaster]
The whole subject of the X rays is opening out wonderfully, Bragg has of course got in ahead of us, and so the credit all belongs to him, but that does not make it less interesting. We find that an X ray bulb with a platinum target gives out a sharp line spectrum of five wavelengths which the crystal separates out as if it were a diffraction grating. In this way one can get pure monochromatic X rays. Tomorrow we search for the spectra of other elements. There is here a whole new branch of spectroscopy, which is sure to tell one much about the nature of an atom.
Letter to his mother (18 May 1913). In J. L. Heilbron (ed.), H. G. J. Moseley: The Life and Letters of an English Physicist 1887-1915 (1974), 205.
The X-ray spectrometer opened up a new world. It proved to be a far more powerful method of analysing crystal structure…. One could examine the various faces of a crystal in succession, and by noting the angles at which and the intensity with which they reflected the X-rays, one could deduce the way in which the atoms were arranged in sheets parallel to these faces. The intersections of these sheets pinned down the positions of the atoms in space.… It was like discovering an alluvial gold field with nuggets lying around waiting to be picked up.… It was a glorious time when we worked far into every night with new worlds unfolding before us in the silent laboratory.
In The History of X-ray Analysis (1943), 9.
There is a place with four suns in the sky—red, white, blue, and yellow; two of them are so close together that they touch, and star-stuff flows between them. I know of a world with a million moons. I know of a sun the size of the Earth—and made of diamond. There are atomic nuclei a few miles across which rotate thirty times a second. There are tiny grains between the stars, with the size and atomic composition of bacteria. There are stars leaving the Milky Way, and immense gas clouds falling into it. There are turbulent plasmas writhing with X- and gamma-rays and mighty stellar explosions. There are, perhaps, places which are outside our universe. The universe is vast and awesome, and for the first time we are becoming a part of it.
Opening paragraph, in 'Introduction' Planetary Exploration (1970), 15.
Treat the patient, not the X-ray
…...
Tungsten, X-rays, and Coolidge form a trinity that has left an indelible impression upon our life and times. The key word in this triad is Coolidge, for his work brought the element tungsten from laboratory obscurity to the central role of the industrial stage and gave the X-ray a central role in the progress of medicine throughout the world.
In National Academy of Sciences, Biographical Memoirs, Vol. 53, 141.
We have now got what seems to be definite proof that an X ray which spreads out in a spherical form from a source as a wave through the aether can when it meets an atom collect up all its energy from all round and concentrate it on the atom. It is as if when a circular wave on water met an obstacle, the wave were all suddenly to travel round the circle and disappear all round and concentrate its energy on attacking the obstacle. Mechanically of course this is absurd, but mechanics have in this direction been for some time a broken reed.
Letter to Margery Moseley (2 Feb 1913). In J. L. Heilbron (ed.), H. G. J. Moseley: The Life and Letters of an English Physicist 1887-1915 (1974), 201.
When I saw the alpha-helix and saw what a beautiful, elegant structure it was, I was thunderstruck and was furious with myself for not having built this, but on the other hand, I wondered, was it really right?
So I cycled home for lunch and was so preoccupied with the turmoil in my mind that didn’t respond to anything. Then I had an idea, so I cycled back to the lab. I realized that I had a horse hair in a drawer. I set it up on the X-ray camera and gave it a two hour exposure, then took the film to the dark room with my heart in my mouth, wondering what it showed, and when I developed it, there was the 1.5 angstrom reflection which I had predicted and which excluded all structures other than the alpha-helix.
So on Monday morning I stormed into my professor’s office, into Bragg’s office and showed him this, and Bragg said, 'Whatever made you think of that?' And I said, 'Because I was so furious with myself for having missed that beautiful structure.' To which Bragg replied coldly, 'I wish I had made you angry earlier.'
So I cycled home for lunch and was so preoccupied with the turmoil in my mind that didn’t respond to anything. Then I had an idea, so I cycled back to the lab. I realized that I had a horse hair in a drawer. I set it up on the X-ray camera and gave it a two hour exposure, then took the film to the dark room with my heart in my mouth, wondering what it showed, and when I developed it, there was the 1.5 angstrom reflection which I had predicted and which excluded all structures other than the alpha-helix.
So on Monday morning I stormed into my professor’s office, into Bragg’s office and showed him this, and Bragg said, 'Whatever made you think of that?' And I said, 'Because I was so furious with myself for having missed that beautiful structure.' To which Bragg replied coldly, 'I wish I had made you angry earlier.'
From transcript of audio of Max Perutz in BBC programme, 'Lifestory: Linus Pauling' (1997). On 'Linus Pauling and the Race for DNA' webpage 'I Wish I Had Made You Angry Earlier.'
When you no longer know what headache, heartache, or stomachache means without cistern punctures, electrocardiograms and six x-ray plates, you are slipping.
While the biological properties of deoxypentose nucleic acid suggest a molecular structure containing great complexity, X-ray diffraction studies described here … show the basic molecular configuration has great simplicity. [Co-author with A.R. Stokes, H.R. Wilson. Thanks include to “… our colleagues R.E. Franklin, R.G. Gosling … for discussion.”]
From 'Molecular Structure of Deoxypentose Nucleic Acids', Nature (25 Apr 1953), 171, No. 4356, 738. (Note: in W.F. Bynum and Roy Porter (eds.), Oxford Dictionary of Scientific Quotations (2005), 226, this quote is listed under Rosalind Elsie Franklin and cited, incorrectly, as from “Rosalind Franklin and R. G. Gosling, 'Molecular Structures of Nucleic Acids: A Structure for Deoxyribose Nucleic Acid', Nature, 1953, 171, 741.” However, the Franklin and Gosling article on p.741 is the second of two pages titled 'Molecular Configuration in Sodium Thymonucleate'.)
Words can be like X-rays, if you use them properly—they’ll go through anything. You read and you’re pierced.
In Brave New World (1932, 1950), 58.
X-rays ... I am afraid of them. I stopped experimenting with them two years ago, when I came near to losing my eyesight and Dally, my assistant practically lost the use of both of his arms.
Quoted in 'Edison Fears Hidden Perils of the X-Rays', New York World (3 Aug 1903), 1.
X-rays will prove to be a hoax.
In Marc J. Madou, Fundamentals of Microfabrication: the Science of Miniaturization (2nd ed., 2002), 325.
X-rays. Their moral is this—that a right way of looking at things will see through almost anything.
Samuel Butler, edited by Geoffrey Keynes and Brian Hill, Samuel Butler’s Notebooks (1951), 282.